Physical mechanisms of vascular system morphogenesis
Participants : Annemiek Cornelissen, Marc Durand
Biological transport networks are essential to the functioning of many organisms. Examples include the vascular system of vertebrates (from aorta to capillary bed) and plants (from roots to leaf venation), the bronchial system and the mycelium of fungal colonies. These self-organizing structures display a number of properties (efficiency, adaptability, resilience) that are highly desirable for technical applications. Understanding their formation and evolution also has obvious medical applications, as many diseases are associated with pathological evolutions of their structure. structure. It is well documented that the growth and remodeling of biological transport networks are influenced by mechanical factors. Using model organisms, the myxomycete Physarum polycephalum and the jellyfish Aurelia aurita, we are investigating the importance of mechanical constraints (emanating from surrounding tissues or internal flows) on the formation and remodeling of biological networks. We are also interested in the self-organizing mechanisms of contractile activity, responsible for the flows generated in these heartless organisms.
Morphogenesis of the canal network of the gastrovascular system of the jellyfish Aurelia aurita
We are studying the morphogenesis of the canal network of the gastrovascular system of the jellyfish Aurelia aurita. We aim to characterize the network on a macroscopic scale. By quantifying growth through daily observations of the network, we have developed algorithms that enable us to trace the growth of the canal network. Using the growth history, we are able to quantitatively assess pattern diversity. We assume that the patterns originate from self-organizing instabilities. The first instability we observe is analogous to crack propagation. The muscles of the sub-umbra contract, generating compression in the endodermal sheet in which the canals develop. Like cracks, hollow, soft canals release compressive stress in the endodermal sheet. To understand how compressive stress is distributed in the endoderm, we observed small deformations due to muscle contractions when the jellyfish lay flat under the microscope, and large deformations during swimming.
Simulation models have enabled us to estimate the stress distribution in the endodermal leaflet around the canals. The position of the maximum stress correlates with the biased bud growth observed in jellyfish. The second instability is analogous to branching instabilities in hydrodynamic systems, such as the well-known Saffman-Taylor experiment, injecting air into oil. When the mobility of the invading phase (air) is greater than the mobility of the displaced phase (oil), the boundary between the phases becomes unstable and the front breaks into fingers. When the ratio of the mobilities of the two phases is low, these fingers can reconnect. An extreme case occurs when one finger breaks off and the neighbouring finger is still connected. It is exactly this time-correlated reconnection that we observe in Aurelia’s channel network.
Contractile activity and tubular network development in P. polycephalum
In its plasmode phase, P. polycephalum is a giant unicellular organism which develops a tubular network in which flows are generated by the active contraction of the membranous layer surrounding the “veins”, making up for diffusive flows which are too slow. However, generating flows over long distances requires the coordination of these contractions, in the form of contraction waves. Throughout its life, the organism is able to remodel its network architecture via local control of the sol-gel transition. To elucidate the coupling mechanisms between contractile activity, flow and network formation (or remodeling), we studied the coordination of contractile activity and network remodeling in specimens confined in chambers of annular geometry whose radius and width can be varied (see figure opposite).
This geometry eliminates any predefined anteroposterior axis of the organism, and blocks growth or migration when the chamber is completely filled. This geometry also makes the system one-dimensional. We have built an original transmission imaging device placed in a humidity- and temperature-controlled chamber. We also developed specific image processing and signal analysis tools to fully characterize the network structure (topology, vein lengths and diameters, conductances) – with unprecedented resolution enabling us to identify several thousand veins – as well as the contraction waves (instantaneous phase, amplitude, wavelength, frequency, phase velocity) and flow rates passing through the network. This set-up enabled us to characterize the various stable contractile modes that could be established in the annular chamber, and their respective lifetimes. These results enabled us to discriminate between the different models of coupling between contractions and flows that exist in the literature. We also highlighted and quantified the long-time reorientation of the network veins along the orthoradial direction.
Read more
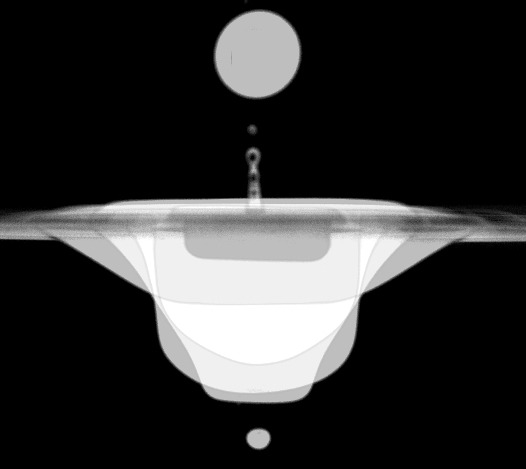
Bubble entrapment by drop impact
Only under certain conditions does a drop falling onto a bath entrap an air bubble. We propose a phenomenological law that describes these bubbling conditions in terms of Froude, Weber, and capillary numbers.Figure. Superimposed images of a drop and the cavity it...
18-month post-doctoral fellowship on pulmonary surfactant
Structure and rheology of pulmonary surfactantResearch The INHALE project, funded by the French National Research Agency (ANR), seeks to investigate the viability of surfactant replacement therapy in adults afflicted with respiratory disorders. Pulmonary surfactant is...
M2/Thesis 2023-2024 internship proposal
Nuclear mechanics as a diagnostic and therapeutic target for glioblastoma Glioblastomas (GBMs) are the most lethal primary brain tumours. The absence of effective therapies is mainly due to tumour invasion and to the resistance of invading cells to treatments such as...
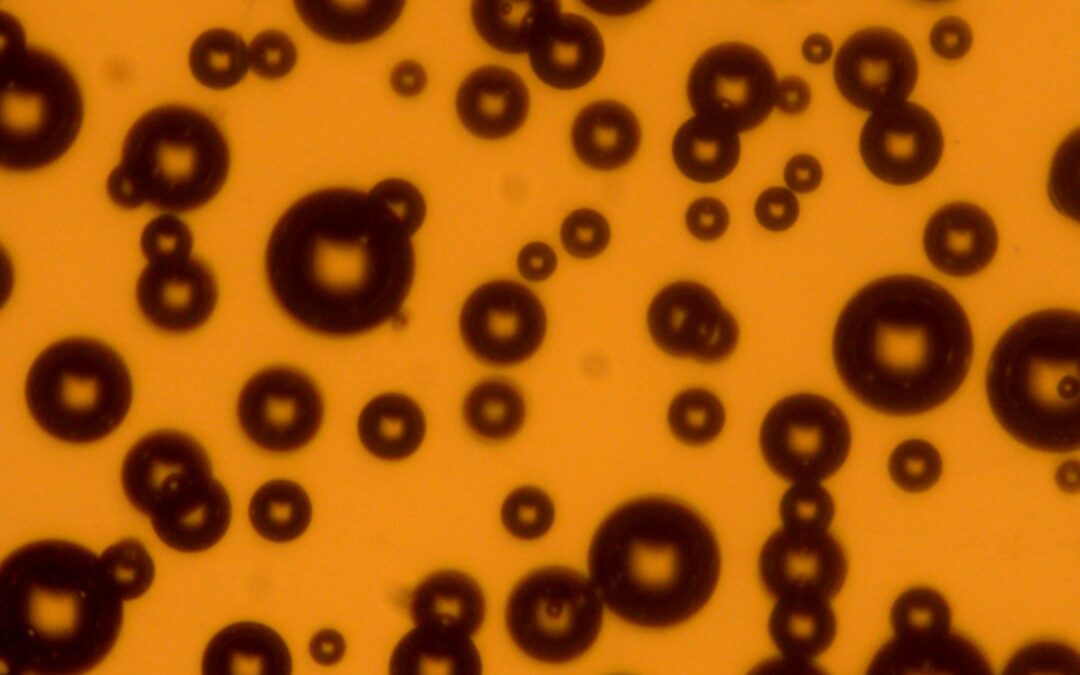
PhD defense. 05/11/2024, Lorijn van der Spek: “Application of mechanical and geometrical constraints of muscle cells to promote differentiation at different scales”
Tuesday, November 5th, Bâtiment Condorcet, 10 rue Alice Domon et Léonie Duquet, 75013 Paris. PhD defense of Lorijn van der Spek Application of mechanical and geometrical constraints of muscle cells to promote differentiation at different scales Supervised by Myriam...